In the world of technology, quantum computing stands as one of the most revolutionary and cutting-edge fields, promising to reshape the landscape of computation as we know it. Unlike classical computing, which operates on bits, quantum computing harnesses the principles of quantum mechanics to manipulate quantum bits, or qubits.
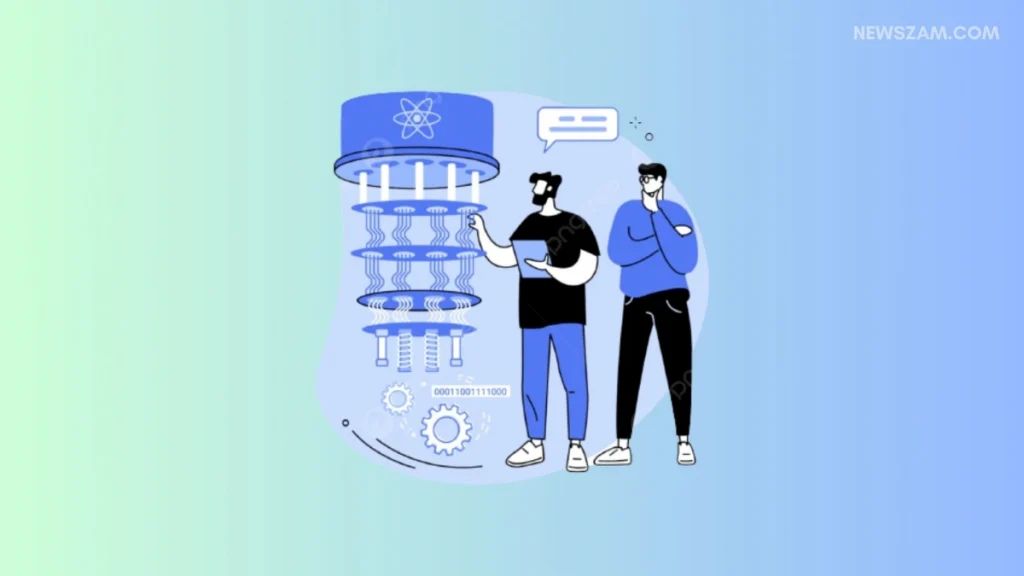
This fundamental distinction opens up entirely new possibilities for solving complex problems that were previously beyond the reach of classical computers. In this article, we will explore the concept of quantum computing and delve into the key differences that set it apart from classical computing.
Understanding Quantum Computing:
Quantum computing is a type of computing that utilizes the principles of quantum mechanics, a branch of physics that describes the behavior of matter and energy at the smallest scales—atoms and subatomic particles. At its core, quantum computing relies on qubits, which can exist in multiple states simultaneously, a phenomenon known as superposition. This property allows quantum computers to perform vast numbers of calculations in parallel, granting them a significant advantage over classical computers for specific types of problems.
Differences from Classical Computing:
Bits vs. Qubits: Classical computers utilize bits, which are binary units of information represented as either 0 or 1. These bits process data sequentially, performing operations one after the other. In contrast, quantum computing uses qubits, which can exist in multiple states simultaneously due to the principle of superposition. This enables quantum computers to process multiple possibilities in parallel, vastly increasing their computational power for certain tasks.
Superposition and Entanglement:
Superposition is a unique characteristic of qubits that allows them to hold multiple states at once. Entanglement is another crucial principle of quantum mechanics, where qubits become linked, and the state of one qubit becomes dependent on the state of another, even when they are far apart. These properties allow quantum computers to perform complex calculations and simulations at speeds exponentially faster than classical computers.
Computational Power:
Quantum computing offers the potential to solve specific problems much faster than classical computers. For example, quantum algorithms, such as Shor’s algorithm, have the capability to factor large numbers exponentially faster than the best-known classical algorithms, which has significant implications for cryptography and data security.
Quantum Parallelism:
Quantum parallelism is a key advantage of quantum computing. It allows quantum computers to explore and evaluate multiple solutions simultaneously, enabling more efficient solutions to certain computational problems.
Quantum Decoherence:
One of the challenges in quantum computing is maintaining the delicate state of qubits. Quantum decoherence refers to the loss of quantum information due to interactions with the external environment, which can lead to errors in computations. Overcoming quantum decoherence is crucial for building practical quantum computers.
Scalability:
Building large-scale, error-resistant quantum computers is a significant engineering challenge. While quantum computing shows great promise, it is still in its early stages, and building reliable, scalable quantum computers remains a formidable task.
How does quantum entanglement play a role in quantum computing?
Quantum entanglement is a fundamental principle of quantum mechanics and plays a crucial role in quantum computing. It is one of the key features that sets quantum computing apart from classical computing and enables quantum computers to perform certain calculations exponentially faster. Let’s explore how quantum entanglement works and its significance in quantum computing:
Understanding Quantum Entanglement:
Quantum entanglement is a phenomenon that occurs when two or more particles become correlated in such a way that the state of one particle is dependent on the state of another, regardless of the distance between them. When particles become entangled, they lose their individuality and form a single quantum system. This means that the measurement of one entangled particle instantly determines the state of the other, no matter how far apart they are, even if they are light-years away.
Role of Quantum Entanglement in Quantum Computing:
- Increased Computational Power: Quantum entanglement enables quantum computers to process information simultaneously across multiple qubits. Unlike classical bits, which can only exist in a state of 0 or 1, qubits can be in a superposition of both 0 and 1 simultaneously. When qubits are entangled, their states become correlated, and a change in one qubit instantly affects the other. This parallelism allows quantum computers to perform many calculations simultaneously, vastly increasing their computational power for certain tasks.
- Quantum Parallelism: Quantum parallelism is a concept derived from quantum entanglement and superposition. Since qubits can exist in multiple states at once, quantum computers can explore and evaluate multiple solutions to a problem simultaneously. This exponentially increases the number of possibilities that can be processed at the same time, providing a significant advantage over classical computers for certain types of computations.
- Quantum Communication: Quantum entanglement also plays a vital role in quantum communication, which is a critical aspect of quantum computing. By entangling qubits and using them as quantum bits to transmit information, it is possible to achieve secure and efficient communication. Quantum entanglement allows for the creation of quantum communication channels, such as quantum teleportation, that enable the transfer of quantum states between remote locations with perfect fidelity.
- Quantum Error Correction: Quantum entanglement is essential for quantum error correction, which is crucial in building reliable quantum computers. Quantum computers are susceptible to errors due to interactions with the environment, known as quantum decoherence. Quantum error correction techniques use entanglement to detect and correct errors without disturbing the quantum information stored in qubits.
Challenges and Implications:
Despite the immense potential of quantum entanglement in quantum computing, there are also challenges associated with maintaining entangled states and mitigating the effects of decoherence. Keeping qubits entangled and stable is a complex task that requires precise control and isolation from external disturbances.
What are the potential applications of quantum computing in various industries?
Quantum computing holds the potential to revolutionize various industries by solving complex problems that are beyond the capabilities of classical computers. Let’s explore the potential applications of quantum computing in the fields of finance, healthcare, and cryptography:
1. Finance:
a. Portfolio Optimization: Quantum computing can efficiently analyze vast amounts of financial data and optimize investment portfolios by considering multiple variables and constraints simultaneously. This could lead to more accurate risk assessment and better-performing investment strategies.
b. Option Pricing and Risk Analysis: Quantum algorithms can tackle complex financial modeling and risk analysis problems, enabling more accurate option pricing, credit risk assessments, and simulations for stress testing.
c. Cryptographic Key Generation: Quantum computing can generate secure cryptographic keys based on the properties of entangled qubits, providing enhanced security for financial transactions and data protection.
2. Healthcare:
a. Drug Discovery: Quantum computing can significantly accelerate the drug discovery process by simulating molecular interactions and identifying potential drug candidates more efficiently. This could lead to the development of novel drugs for various diseases.
b. Genomics and Personalized Medicine: Quantum computing can analyze vast genomic datasets and predict disease susceptibility, optimize personalized treatment plans, and identify potential genetic markers for specific medical conditions.
c. Medical Imaging: Quantum computing can enhance medical imaging techniques, such as MRI and CT scans, by processing large amounts of image data and improving image reconstruction and analysis.
3. Cryptography:
a. Breaking Cryptographic Protocols: Quantum computers have the potential to break widely used cryptographic protocols, such as RSA and ECC, which rely on the difficulty of factoring large numbers. Shor’s algorithm, a quantum algorithm, can efficiently factor large numbers, rendering classical encryption vulnerable.
b. Quantum Key Distribution (QKD): Quantum computing can offer solutions to improve the security of communication through Quantum Key Distribution. QKD leverages quantum entanglement to ensure secure key exchange between parties, providing protection against eavesdropping.
c. Post-Quantum Cryptography: Quantum computing can also aid in the development of post-quantum cryptographic algorithms that are resistant to quantum attacks. These cryptographic schemes will play a crucial role in securing data against quantum threats.
It is important to note that while quantum computing offers great promise, it is still in its early stages of development, and large-scale, error-resistant quantum computers are yet to be realized. The practical implementation of quantum computing applications in various industries will depend on overcoming technical challenges, maintaining quantum coherence, and ensuring error correction.
Conclusion:
Quantum computing represents a profound shift in the world of computing, offering the potential to revolutionize problem-solving and computational capabilities.
While classical computing remains the workhorse for many everyday tasks, quantum computing’s ability to harness the power of superposition and entanglement opens up new possibilities for tackling complex problems in fields such as cryptography, drug discovery, optimization, and artificial intelligence.
As research and development in quantum computing continue to progress, it holds the promise of transforming the way we approach the most challenging problems of our time.
Leave a Reply